Cosmological simulations
Chemodynamical simulations (Kobayashi)
We are simulating the evolutionary history of the Universe across cosmic time using a self-consistent 3D hydrodynamical code (Kobayashi et al. 2007). This code is based on Gadget-3, where the Smoothed Particle Hydrodynamics (SPH) method is used to describe the hydrodynamics. We also include detailed physical processes undergone by atomic matter: namely, star formation, feedback from supernovae and active galactic nuclei (AGN; Taylor & Kobayashi 2014), and chemical enrichment from core-collapse and thermonuclear supernovae, and AGB stars (Kobayashi 2004; Kobayashi et al. 2011). These simulations are capable of comparison with observations of nearby and distant galaxies.
Cosmic chemical enrichment
We have predicted the time/redshift evolution of the spatial distributions of elements, which are consistent with observations of star forming galaxies at high redshift (z~2) and early-type galaxies at low redshift (z~0). Chemical enrichment is dependent on the environment and - as is well known - metallicity varies even inside galaxies.
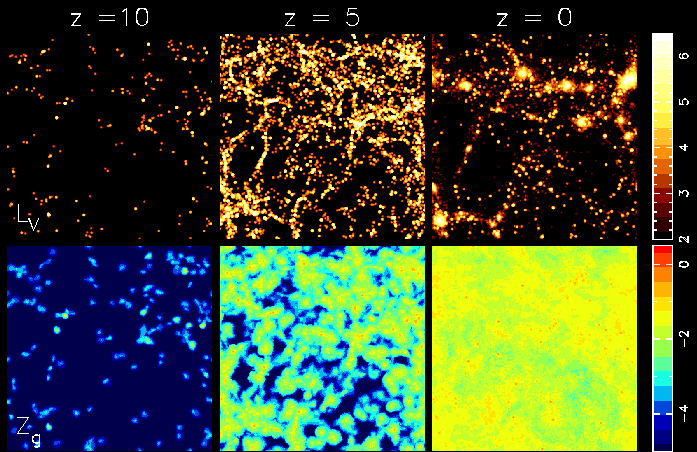
Supernova feedback
Supernovae eject not only thermal energy that serves to suppress star formation, but they also produce heavy elements to enhance star formation. Supernova feedback, in particular hypernova feedback (Kobayashi et al. 2007), generates galactic winds from present-day less-massive galaxies and heavy elements are ejected from galaxies into the intergalactic medium.
AGN feedback
Feedback from active galactic nuclei (AGN) works more efficiently in massive galaxies (Croton et al. 2004). We have introduced a new model for seeding black holes motivated by the formation of the first stars in our cosmological simulations (Taylor & Kobayashi 2014), and showed better agreement with the observed down-sizing phenomena (Taylor & Kobayashi 2015a), and AGN-driven metal-enhanced outflows from massive galaxies (Taylor & Kobayashi 2015b).
Horizon-AGN & New Horizon simulations (Kaviraj)
A significant recent advance has been the advent of hydro-dynamical cosmological simulations, which offer survey-scale predictions of the evolution of galaxy properties over cosmic time. When calibrated against the array of multi-wavelength observational data now available, these models offer powerful insights into the processes that drive galaxy evolution, especially in the still-poorly-understood high-redshift Universe.
Horizon-AGN is a cosmological hydro-dynamical simulation in a comoving volume of 100 Mpc/h, with 10243 dark matter particles in a Planck cosmology, performed using the adaptive mesh refinement code RAMSES (Teyssier, 2002). The adaptive mesh refinement technique enables zooming in with more resolution elements in regions of interest (e.g. structures such as cosmic filaments, galaxies, giant molecular clouds, etc), with the Riemann-based hydro-dynamical solver employed by the code being very efficient at capturing sharp discontinuities in the gas flow and gas instabilities that spontaneously arise in a cosmic turbulent medium. The simulation includes hydrodynamics and gas cooling and heating, with sub-grid models employed for the treatment of the physics of galaxy evolution: star formation, feedback from stars (stellar winds, Type II and Type Ia supernovae), chemical enrichment from six species (O, Fe, C, N, Mg, Si) and feedback from active galactic nuclei. The minimum cell size is 1 kpc, constant in physical length.
NewHorizon is a zoom-in with a volume of (16 Mpc)3 within Horizon-AGN, that simulates an average density region of the larger simulation. It offers a resolution of up to 34 pc, allowing us to capture the multi-phase nature of the interstellar medium in unprecedented detail.
At Hertfordshire, we are using Horizon-AGN and NewHorizon, in concert with survey data from ground and space-based instruments to probe key open questions in galaxy evolution, particularly in the early Universe: e.g. at what redshifts did the Hubble sequence emerge? at what epochs and via what processes were the primordial spheroids built? what processes dominated the build-up of stellar mass and black holes around the epoch of peak star formation? what role did AGN have in triggering or suppressing early star formation?
A description of the two simulations can be found in Kaviraj et al. 2017 and Dubois et al. 2021.
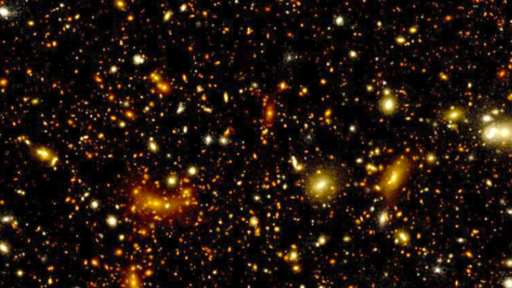
L-Galaxies simulation (Yates)
L-Galaxies is a cosmological-scale, semi-analytic simulation of galaxy evolution, developed by researchers at CAR and their international team of collaborators.
The key advantage of L-Galaxies is its efficiency. The semi-analytic method divides-up each galaxy into a set of “homogeneous spatial components”, rather than using computational particles or cells. This approach enables L-Galaxies to simultaneously model millions of galaxies across all cosmic time within very large cosmological boxes >(500 Mpc/h)3 many thousands of times quicker than full hydrodynamical simulations of comparable size.
The main focus of research with L-Galaxies at CAR is on galaxy chemical evolution (GCE). The simulation contains a world-leading GCE model which includes every element in the periodic table. This model tracks the birth of stars and evolution of star, and the distribution of the newly-synthesised chemical elements they produce into the surrounding gas via supernovae and other events. This GCE model has been used to study chemical enrichment in various types of galaxy across cosmic time, including galaxy clusters (Yates et al. 2017), star-forming galaxies (Yates et al. 2021a), and the Universe as a whole (Yates et al. 2021b). Most recently, dust production and chemical enrichment from binary stars have been incorporated (Yates et al. 2024).
Future work with L-Galaxies will look at the chemical composition of the first galaxies to form, as well as the complex chemistry found in our own Milky Way (see our Galactic astronomy page). L-Galaxies will also be used along with other simulations to study habitability across the Universe in greater detail than ever before. With the inclusion of chemical elements, dust, and molecules into the simulation, we will be able to predict when the Universe first developed the conditions required for life, and in which galaxies we are most likely to find it.
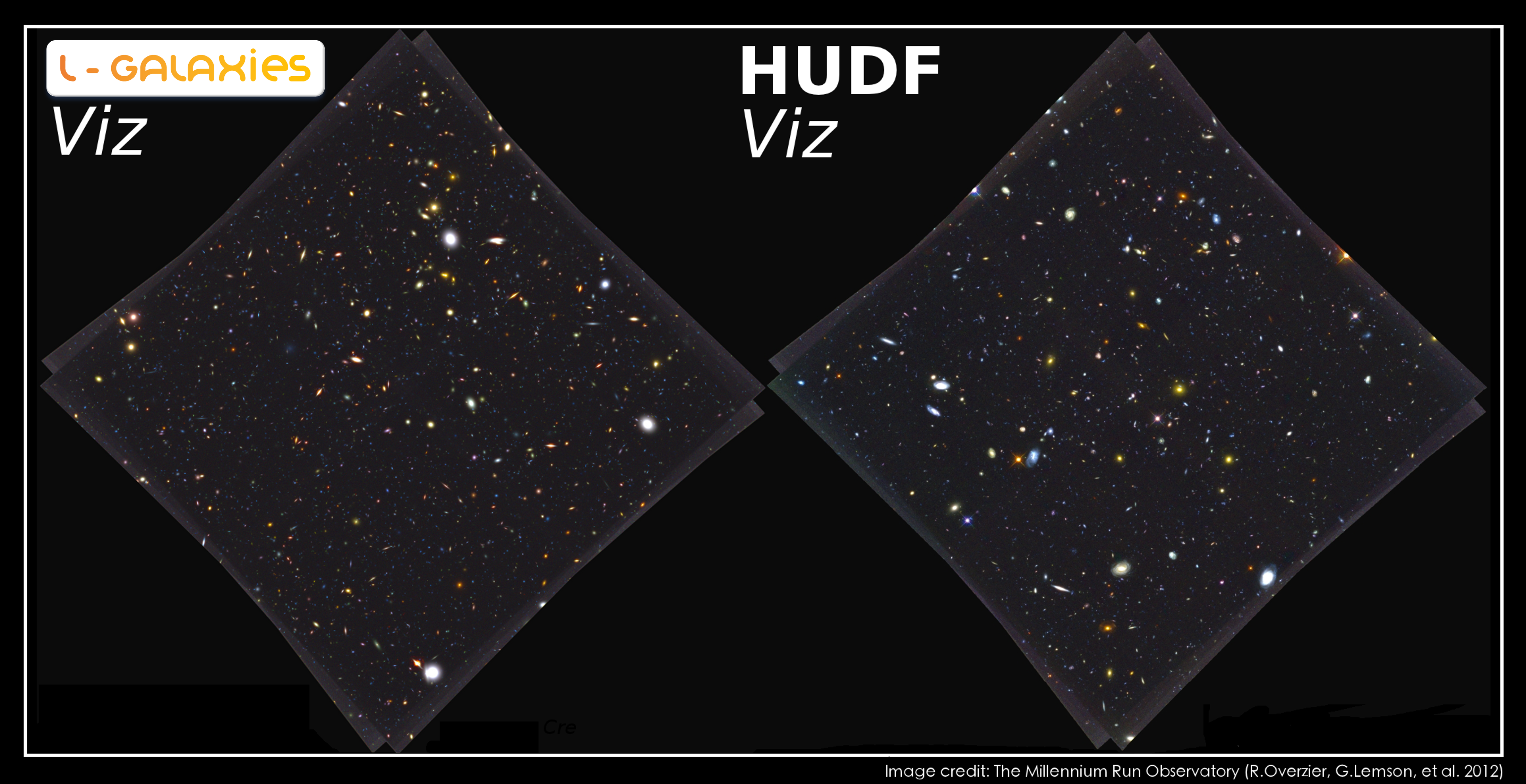