Active Galactic Nuclei (AGN)
(Alston, Drake, Gürkan, Hardcastle, Krause, Stevens, Walton)
Active galaxies (AGN) are bright regions at the centres of a small minority of galaxies, powered by accretion onto the galactic-centre supermassive black hole.
Radio-quiet AGN
RQAGN are galaxies with supermassive black holes (SMBHs) that emit energy radiatively across the electromagnetic spectrum. These AGN feature typical components such as an accretion disk, an X-ray corona, high-excitation emission lines, and a mid-IR torus. These objects also produce radio emission, the origin of which is debated; it may arise from small-scale jets, star formation, or both. Our work focuses on understanding the source of radio emission, the AGN/galaxy connection, the broad-band spectral energy distributions, and the feedback from these sources using radio surveys such as EMU and LOFAR.
Radio-loud AGN
The most poorly understood class of active galaxies is the small minority of radio-loud objects (including radio galaxies and radio-loud quasars), in which the active nucleus emits twin relativistic jets of highly energetic particles (electrons and positrons, and maybe heavier particles too). These jets can propagate out to distances of megaparsecs and the material that flows through them inflates large `lobes' of energetic plasma which radiates by the synchrotron process. Radio observations give insight into their complex and disparate structure but cannot on their own tell us much about quantities that interest us as physicists (energetics, internal physical conditions, dynamics and so on). In the past two decades, using the new generation of imaging X-ray telescopes (Chandra and XMM-Newton), it has become possible to make observations that probe these physical conditions directly. We are interested in the following aspects:
- The physics of the active nucleus, particularly the nature of the accreting material and 'unified models' that seek to explain the different appearance of active nuclei (observation and theory)
- Physical conditions, bulk speeds and particle acceleration in the large-scale jets and the lobes that they form (observation and modelling)
- The impact of the large-scale jets on their environment (observation and modelling)
- The acceleration of ultra-high-energy cosmic rays (observation, theory and modelling).
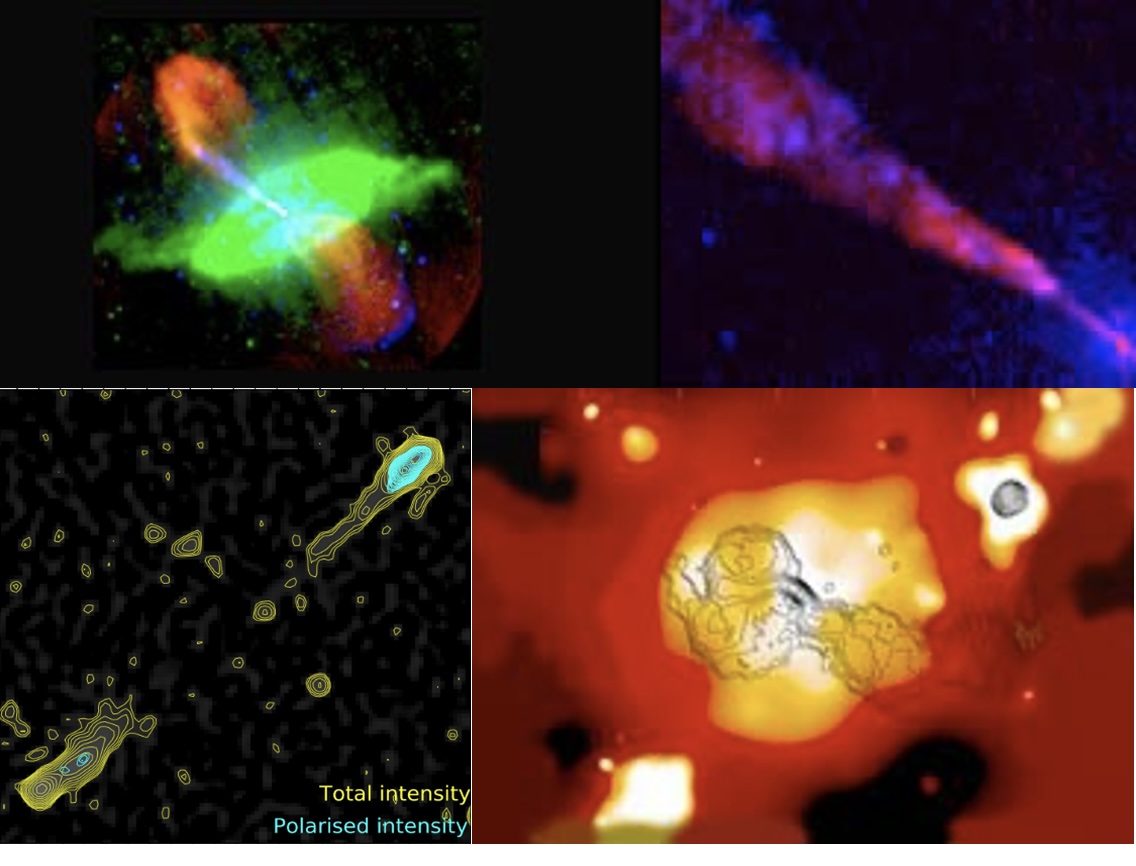
X-ray and gamma-ray emission from the radio sources themselves
Radio sources can emit X-rays (and optical light) via the synchrotron process, and observations of this process, pioneered by us, have given us new insights into particle acceleration in the jets in low-power radio galaxies and in the shocks at the terminations of high-power jets: the electrons emitting X-rays have very short radiative lifetimes, so that X-ray emission is a diagnostic of truly local particle acceleration.
However, the most important process for understanding physical conditions in radio galaxies is the related inverse-Compton process, in which relativistic electrons scatter low-energy photons into the X-ray band. This process is vital because, if we know the properties of the parent photon population, as we typically do, we can infer the properties of the relativistic electrons, such as their energies and (most importantly) their density. This information cannot be obtained directly from observations of synchrotron radiation, though such observations (e.g. with JVLA, LOFAR, EMU, POSSUM) are a crucial part of the process. By combining the information from inverse-Compton and synchrotron processes, we can infer the internal energy density and pressure in components of the radio source, which allows us to make quantitative statements about their dynamics and energetics. We have used inverse-Compton emission extensively to model the dynamics of hot spots and lobes in powerful radio sources, and to map the variations of magnetic field strength and particle energy densities throughout the radio lobes. Our work in this area relies heavily on in-house numerical modelling codes.
For X-ray synchrotron sources, inverse-Compton emission will only be detectable in the gamma-ray; we have worked on modelling TeV gamma-ray emission from these sources in preparation for the Cerenkov Telescope Array (CTA).
The interactions of radio galaxies and their environments
As radio lobes are collections of highly energetic particles, it has been clear for many years that there must be a medium outside them that prevents them from expanding rapidly and disappearing. The only phase of the intergalactic medium that has pressure sufficient to confine radio lobes is the hot, X-ray emitting gas that lies in the potential wells of massive galaxies, groups of galaxies and clusters. Our work with the new X-ray telescopes has shown that there is indeed an intimate relationship between the X-ray emitting material and the radio source: the radio source is both shaped by the X-ray material and in turn does work on it, which is likely to be energetically important in groups and clusters. The impact of AGN activity on the hot, X-ray emitting gas in galaxies, groups and clusters is now understood to be one of the major uncertainties in our understanding our how the Universe has evolved to its present state. In particular, the outbursts from radio-loud AGN appear to be a crucial ingredient for models of galaxy evolution and of the co-evolution of galaxy bulges and black holes, as well as for understanding the observed properties of the intracluster medium. It is also essential that the effects of AGN outbursts on scaling relations between X-ray observables and cluster mass should be understood in order to test structure formation models and to use galaxy clusters as cosmological probes. In recent years our work in this area has focussed on observation and magnetohydrodynamical modelling of interactions between powerful radio galaxies and their environment. The ultimate goal is to derive a robust measurement of the power being put into the hot phase by the radio galaxy without resorting to expensive X-ray observations, and it has been possible to show that this roughly balances the X-ray cooling rate for AGN in the LOFAR surveys.
High Energy Astrophysics: X-rays and Accretion
(Alston, Walton)
Most major galaxies are now expected to host a supermassive black hole (SMBHs) at their centre. When these black holes are actively accreting gas from their surroundings they emit copious amounts of radiation across the entire electromagnetic spectrum, and are referred to as 'active galactic nuclei' (AGN). The innermost regions of these systems are particularly extreme, as they are both highly luminous and extremely compact, as well as being regions of extremely strong gravity. Understanding these regions holds the key to understanding the complex physics of accretion, which dictates both how black holes grow and how they deposit vast amounts of energy back into their surroundings via both radiation and accretion-driven outflows (i.e. winds and jets). Remarkably, these 'feedback' processes can actually carry enough energy for these SMBHs to be able to significantly influence the evolution of their host galaxies!
Much of the emission from these innermost regions emerges in the X-ray band, and at Hertfordshire we study these extreme environments using world leading X-ray telescopes such as XMM-Newton, Swift, Chandra and NuSTAR. We use both spectroscopic and variability studies to try and understand the accretion flows in these systems and how they evolve with accretion rate, and in turn place observational constraints on fundamental properties of the black holes themselves (mass and spin) as well as the impact they can have on their surroundings. We are also preparing for future X-ray facilities, such as ESAs upcoming Athena observatory.
In addition, we also use many of the same facilities and techniques to study X-ray binaries as well as AGN. These are systems in which a compact stellar remnant (typically a black hole or a neutron star left behind by a star at the end of its lifetime) is in a binary system with a 'normal' companion star, and is actively feeding from it. In particular, we study the most extreme members of this population, referred to as 'ultraluminous X-ray sources' (ULXs), which are rare and thus primarily tend to be found in external galaxies. Remarkably, we now know that a small number of these sources are powered by neutron stars that have found a way to significantly surpass their Eddington limits (by factors of up to 500!). We are trying to understand just how these neutron stars are able to reach such extreme luminosities (which may be relevant for rapidly growing SMBHs in the early Universe), as well as the contribution that such neutron stars may make to the broader ULX population.
Radio-Selected Populations of Galaxies and AGN out to High Redshift
(Drake, Gürkan, Hardcastle, Mingo, Smith)
The International LOFAR Telescope has unlocked uncharted parameter space over the past decade by detecting extragalactic sources at very low frequencies with unprecedented sensitivity and speed. LOFAR’s flagship extragalactic survey, LoTSS (“The LOFAR Two- Metre-Sky-Survey”; Shimwell+19, 22) offers us a unique opportunity to select large samples of galaxies and AGN on the basis of their synchrotron radiation, which traces both star formation and AGN activity, making LOFAR-selected targets arguably the most powerful approach to examine the population of all ‘active’ extragalactic sources. A second crucial ingredient for our understanding, is the ability to follow-up sources with complete optical spectroscopy to determine the physical nature of each object we detect – and so Hertfordshire’s leadership of the WEAVE-LOFAR survey (P.I. Smith) which will do exactly this, gives us a unique toolkit to answer some of the most exciting open questions in modern astronomy.
For instance, how do the AGN and star-forming galaxy populations co-evolve from their formation at early times to the population of objects we see in the Universe today? By selecting objects based on their radio emission we are unbiased by the selection effects (most importantly: dust!) that hamper many studies of the population. Our team have also developed new spectroscopic techniques to classify sources, and cleanly select subsets of objects to examine their evolution across cosmic time. In particular, with new LOFAR observations of the Northern Galactic Pole (NGP), and together with the highest quality FIR observations of the northern sky, we are examining what fraction of SMBH growth is obscured by dust and gas, and how this may change over cosmic time. By tackling these exciting questions, systematically and with world-leading datasets, we also work towards answering one of the largest outstanding mysteries - what processes gave rise to the birth of the first supermassive black holes? As the perfect complement to population studies at lower redshifts, we are also involved in analysing observations of individual quasars in the early Universe, representing the most luminous class of AGN, thought to serve as signposts of early galaxy formation. Using data from the panoramic IFU “MUSE” on the VLT, and observations from JWST, we are examining how these powerful objects are growing so rapidly, and how they influence the population of galaxies living in their vicinity.
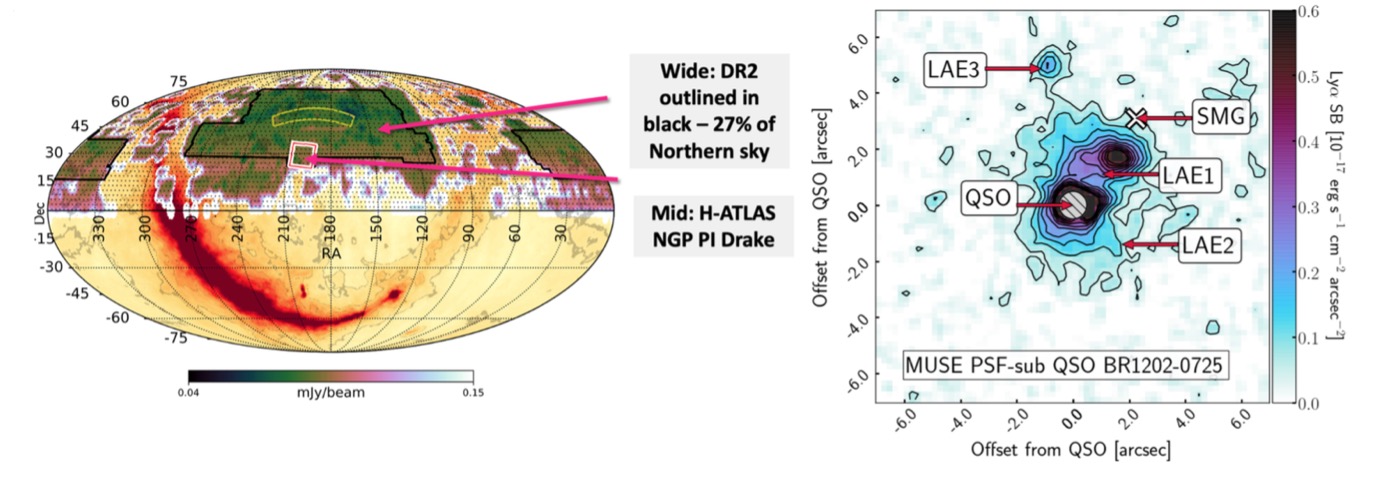